Introduction
Matter is the foundation of everything we interact with in the physical world. It has a vast array of properties that scientists have meticulously studied over centuries. These properties help us understand, classify, and manipulate the materials around us. The properties of matter are typically divided into two broad categories: physical properties and chemical properties. Each of these categories contains several specific properties that can be observed, measured, and analyzed to provide insight into the behavior and characteristics of substances.
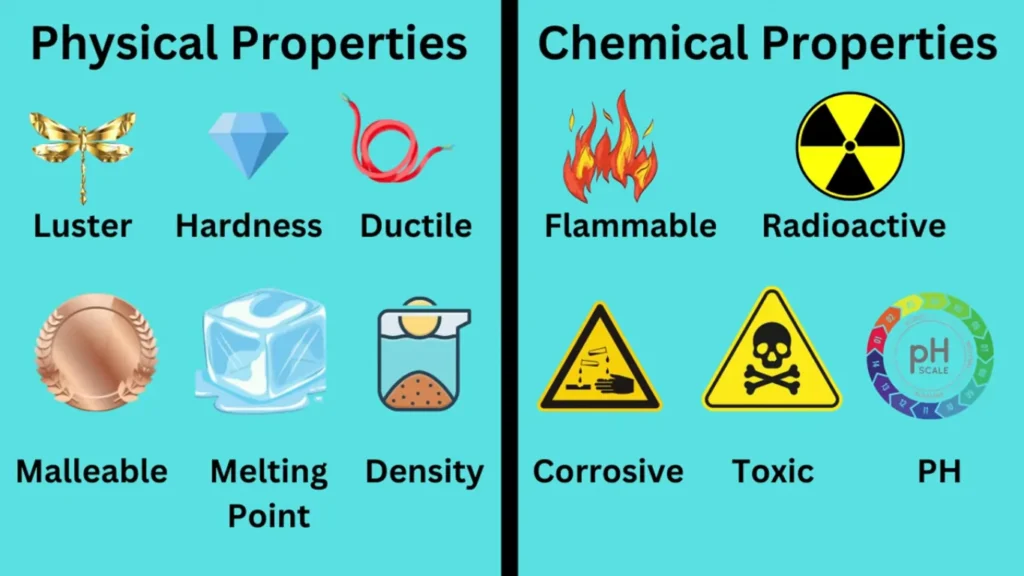
Table of Contents
In this comprehensive exploration, we will delve into the definition of matter, examine the various physical and chemical properties of matter, and discuss the thermal properties, phase changes, and how matter can undergo physical and chemical changes. We will also look at the distinction between intensive and extensive properties and discuss the importance of SI units in measuring physical quantities.
What is Matter?
Matter is defined as anything that has mass and occupies space. This definition encompasses all physical objects, substances, and materials in the universe. Matter is composed of microscopic particles called atoms, which are the building blocks of all substances. These atoms combine in various ways to form molecules, which in turn make up the objects we see, touch, and interact with daily.
Matter exists in three primary states: solid, liquid, and gas. Each of these states has distinct characteristics based on the arrangement and behavior of the atoms or molecules within them. For example:
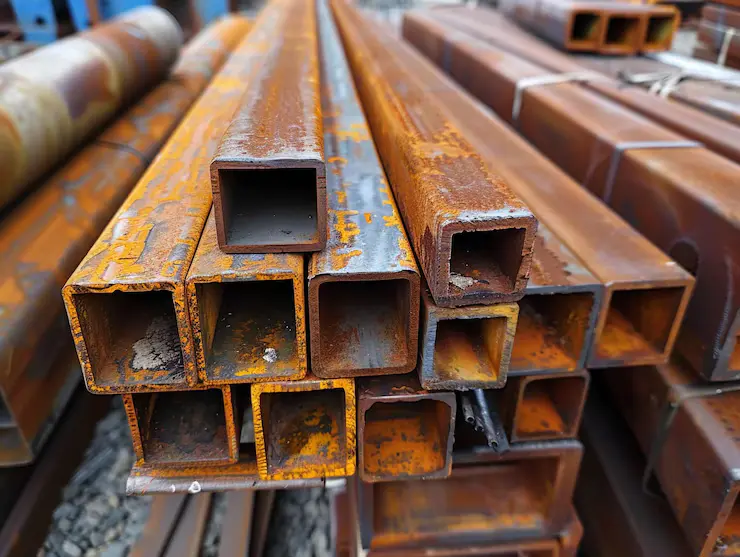
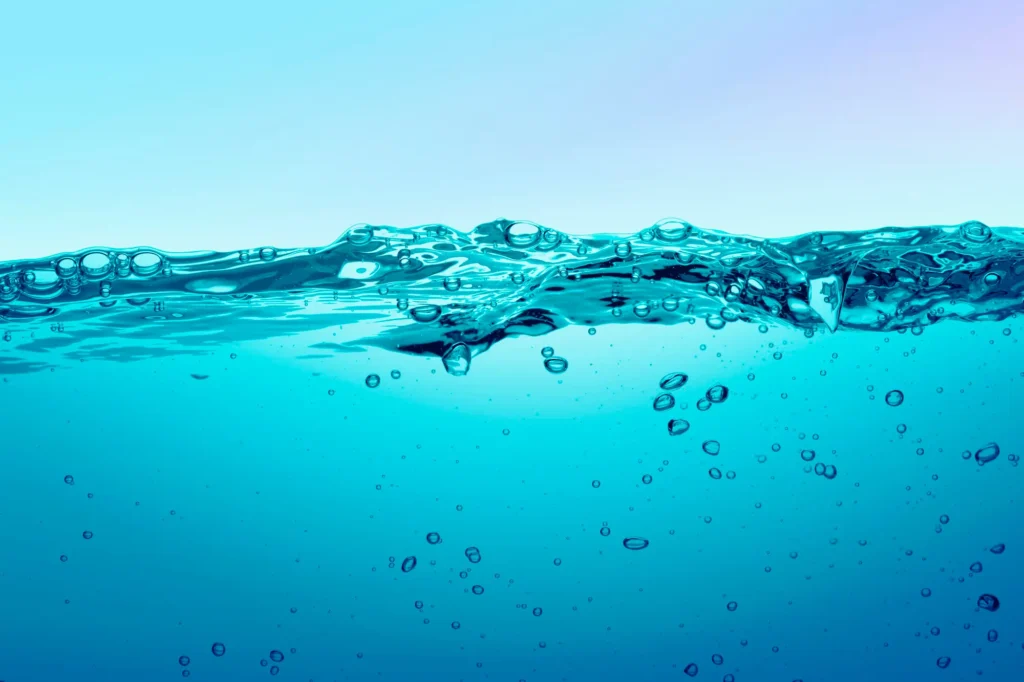
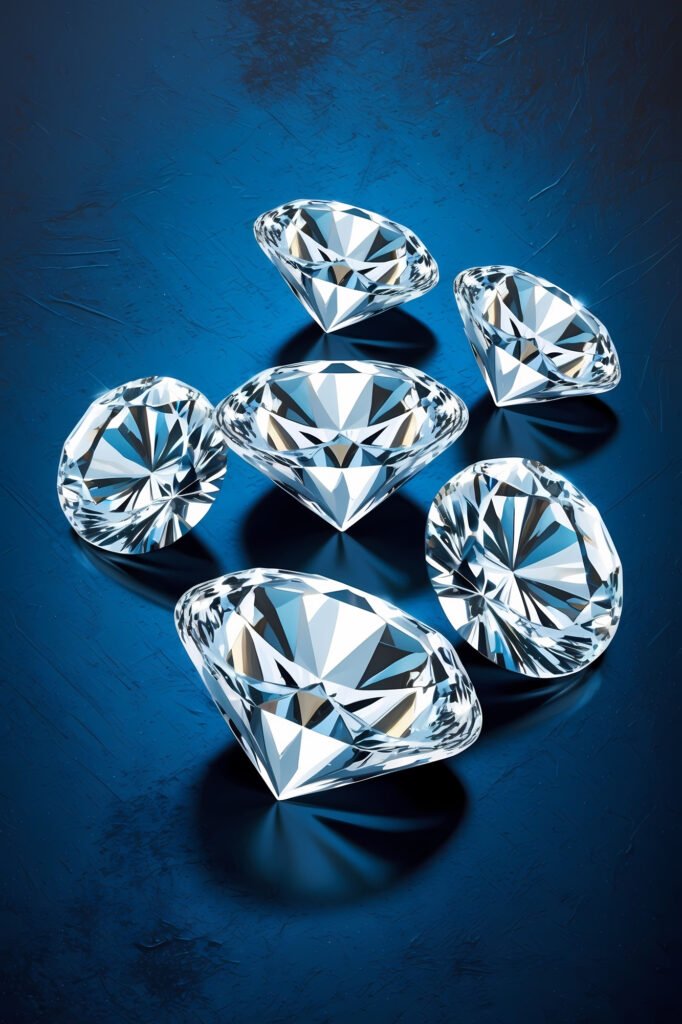
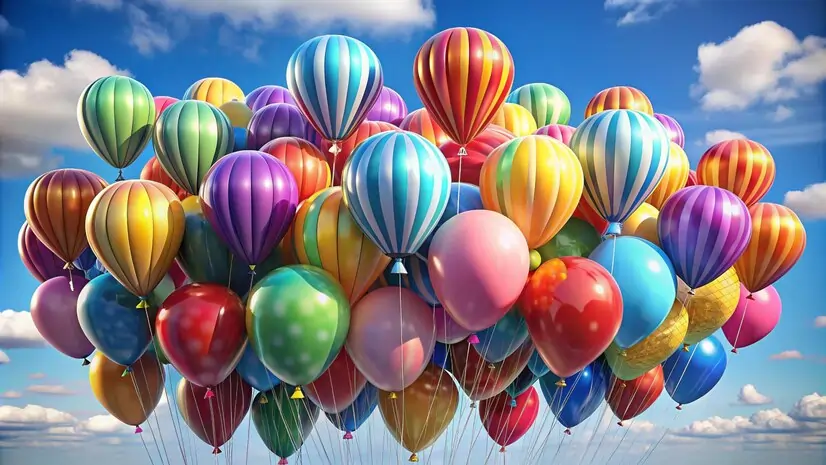
- Solids have a fixed shape and volume due to the closely packed arrangement of their atoms or molecules. This state is characterized by strong intermolecular forces that hold the particles in a rigid structure.
- Liquids have a fixed volume but take the shape of their container. The particles in a liquid are less tightly packed than in a solid, allowing them to flow and move past one another while still maintaining a definite volume.
- Gases have neither a fixed shape nor a fixed volume. The particles in a gas are widely spaced and move freely, filling the entire volume of their container.
Everything in the universe, from the smallest grain of sand to the largest star, is made up of matter. Understanding the properties of matter is crucial to understanding the physical world and the processes that govern it.
Physical Properties of Matter
Physical properties are characteristics of matter that can be observed or measured without altering the substance’s identity or chemical composition. These properties are typically used to describe and classify materials based on their observable and measurable traits. Below, we discuss some of the most important physical properties of matter in detail.
Density
Density is a measure of how much mass is contained within a given volume of a substance. It is expressed mathematically as:
$$
\text{Density} (\rho) = \frac{\text{Mass} (m)}{\text{Volume} (V)}
$$
Density is a critical property that helps us determine whether an object will float or sink in a fluid. For example, an object with a density greater than that of water (1 g/cm³) will sink, while an object with a lower density will float. This principle is applied in various fields, such as shipbuilding and the design of buoyant materials.
Color
Color is a physical property that is easily observable and often used to identify substances. The color of a substance is determined by the wavelengths of light it reflects or absorbs. For instance, the blue color of the sky is due to the scattering of shorter wavelengths of light by the atmosphere, while the green color of leaves is due to the absorption of red and blue light by chlorophyll, a pigment found in plants.
Hardness
Hardness is a measure of a material’s resistance to deformation, scratching, or indentation. It is a crucial property in materials science and engineering, as it determines the suitability of a material for various applications. For example, diamond is known for its exceptional hardness and is often used in cutting tools and abrasives.
Melting and Boiling Points
The melting point of a substance is the temperature at which it changes from a solid to a liquid. Conversely, the boiling point is the temperature at which a liquid changes into a gas. These points are specific to each substance and are used to identify and characterize materials. For instance, water has a melting point of 0°C and a boiling point of 100°C at standard atmospheric pressure.
Electrical Conductivity
Electrical conductivity is a measure of a material’s ability to conduct electric current. Materials with high conductivity, such as metals (e.g., copper and silver), are used in electrical wiring and components, while materials with low conductivity, such as insulators (e.g., rubber and glass), are used to prevent the flow of electricity.
Malleability and Ductility
Malleability refers to the ability of a substance to be deformed under compressive stress, such as being hammered or rolled into thin sheets. Gold, for example, is highly malleable and can be shaped into thin sheets without breaking.
Ductility is the ability of a substance to be drawn into thin wires. Copper is a prime example of a ductile material, making it ideal for use in electrical cables.
Odor
Odor is a physical property related to the sense of smell. Different substances emit different odors due to the volatile compounds they release. For example, the pungent smell of vinegar is due to the presence of acetic acid, while the fresh smell of oranges is due to limonene.
Temperature
Temperature is a measure of the average kinetic energy of the particles in a substance. It is an essential physical quantity used to describe the thermal state of matter. Temperature is measured in various units, including Celsius (°C), Kelvin (K), and Fahrenheit (°F). The temperature of a substance can influence its physical state, chemical reactivity, and other properties.
Chemical Properties of Matter
Chemical properties are characteristics of a substance that can only be observed or measured during a chemical reaction, in which the substance undergoes a transformation into a different substance. These properties are crucial for understanding how substances interact with each other and the conditions under which these interactions occur.
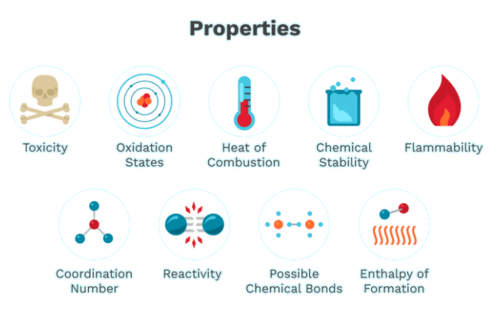
Reactivity
Reactivity refers to the tendency of a substance to undergo chemical reactions, either by itself or with other substances. Reactivity is a key factor in determining the chemical behavior of a substance. For example, potassium is highly reactive, especially when it comes into contact with water. When a small piece of potassium is dropped into water, it reacts violently, producing hydrogen gas and heat, which can ignite the hydrogen, causing an explosion.
Acidity and Basicity
Acidity is a chemical property that describes a substance’s ability to donate protons (hydrogen ions, H⁺) in a chemical reaction. Substances with high acidity are known as acids, and they can react with bases to form salts and water. For example, hydrochloric acid (HCl) is a strong acid that reacts with sodium hydroxide (NaOH), a base, to produce sodium chloride (table salt) and water.
Basicity, on the other hand, refers to a substance’s ability to accept protons. Substances with high basicity are known as bases. An example of a base is ammonia (NH₃), which can accept a proton to form the ammonium ion (NH₄⁺).
Flammability
Flammability is the ability of a substance to burn or ignite in the presence of oxygen. This property is particularly important in the context of fire safety and the handling of combustible materials. For example, wood is a highly flammable material that burns readily when exposed to an open flame, producing heat, light, and various combustion products such as carbon dioxide and water vapor.
Toxicity
Toxicity is a measure of the harmful effects a substance can have on living organisms. Toxic substances can cause a wide range of adverse effects, from mild irritation to severe health problems or even death. Mercury and lead, for example, are highly toxic metals that can cause serious neurological damage if ingested or inhaled.
Heat of Combustion
The heat of combustion is the amount of heat energy released when a substance undergoes complete combustion with oxygen. This property is important in understanding the energy content of fuels. For example, methane (CH₄) has a high heat of combustion, making it an efficient fuel for heating and power generation.
Thermal Properties of Matter
Thermal properties of matter are related to how substances respond to changes in temperature and the transfer of heat. Understanding these properties is essential for applications ranging from industrial processes to everyday activities such as cooking and heating.
Heat Capacity
Heat capacity (C) is the amount of heat energy required to raise the temperature of a substance by 1 degree Celsius or 1 Kelvin. It is an indicator of a substance’s ability to store thermal energy. For example, water has a high heat capacity, which means it can absorb a large amount of heat without a significant increase in temperature. This property is why water is often used as a coolant in various industrial and biological systems.
Specific Heat
Specific heat (c) is the heat capacity per unit mass of a substance. It is a crucial property in understanding how different materials respond to heat. For instance, sand has a lower specific heat than water, which is why beaches get much hotter than the ocean on a sunny day.
Thermal Conductivity
Thermal conductivity is a measure of a material’s ability to conduct heat. Materials with high thermal conductivity, such as metals (e.g., copper and aluminum), transfer heat quickly, making them ideal for use in cooking utensils and heat exchangers. In contrast, materials with low thermal conductivity, such as wood or plastic, are used as insulators to prevent heat loss.
Phase Changes
Phase changes refer to the transitions between different states of matter (solid, liquid, gas) due to changes in temperature or pressure. These changes are associated with the absorption or release of heat energy. The main types of phase changes include:
- Melting: The transition from solid to liquid. For example, ice melts to form water at 0°C.
- Boiling: The transition from liquid to gas. Water boils at 100°C under standard atmospheric pressure.
- Condensation: The transition from gas to liquid. For example, water vapor in the air condenses to form dew on cool surfaces.
- Sublimation: The direct transition from solid to gas without passing through the liquid state. A common example of sublimation is the transformation of dry ice (solid carbon dioxide) directly into carbon dioxide gas.
- Deposition: The direct transition from gas to solid without passing through the liquid state. Frost formation on cold surfaces is an example of deposition.
Melting and Boiling Points
The melting point and boiling point of a substance are specific temperatures at which it undergoes a phase change. These temperatures are characteristic of each substance and are used to identify and classify materials. For example, the melting point of gold is 1,064°C, while the boiling point of mercury is 356.7°C.
Evaporation and Condensation
Evaporation is the process by which a liquid turns into a gas at temperatures below its boiling point. This process occurs at the surface of the liquid and is responsible for phenomena such as the cooling effect of sweat evaporating from the skin.
Condensation is the reverse process, where a gas turns into a liquid. This process releases heat and is commonly observed when water vapor in the air condenses into droplets on a cold surface, such as the formation of water droplets on the outside of a cold glass of water.
Sublimation and Deposition
Sublimation is a phase change where a solid directly transforms into a gas without passing through the liquid phase. This process is used in various industrial applications, such as freeze-drying food and purifying chemicals.
Deposition is the opposite process, where a gas directly transforms into a solid. An example of deposition is the formation of frost on cold surfaces during winter.
Intensive and Extensive Properties of Matter
The properties of matter can be further classified into intensive and extensive properties. This classification is based on whether the property depends on the amount of matter present.
Intensive Properties
Intensive properties are those that do not depend on the amount of matter present. These properties are inherent to the material itself and remain constant regardless of the sample size. Examples of intensive properties include:
- Density: The density of a substance is independent of the amount of material present. For instance, the density of gold remains the same whether you have a small nugget or a large bar.
- Temperature: The temperature of a substance is an intensive property. If you have a glass of water at 25°C, the temperature remains 25°C whether you have one glass or ten glasses of water.
- Pressure: Pressure is another intensive property that is independent of the quantity of matter. The pressure exerted by a gas in a container is the same regardless of the volume of gas, assuming the temperature and volume of the container remain constant.
Extensive Properties
Extensive properties are those that depend on the amount of matter present. These properties change when the size of the sample changes. Examples of extensive properties include:
- Mass: The mass of a substance is directly proportional to the amount of material present. For example, the mass of a gold bar is greater than the mass of a gold nugget.
- Volume: The volume of a substance is another extensive property. The volume of water in a large container is greater than the volume of water in a small container.
- Total energy: The total energy of a system, including kinetic and potential energy, is an extensive property. If you have a larger quantity of a substance, it will contain more total energy than a smaller quantity of the same substance.
Physical and Chemical Changes
Matter can undergo changes that alter its physical state or chemical composition. These changes are classified into physical changes and chemical changes.
Physical Changes
Physical changes are changes that affect the state or appearance of matter but do not alter its chemical composition. These changes are usually reversible. Examples of physical changes include:
- Melting: When ice melts, it changes from a solid to a liquid, but the chemical composition (H₂O) remains the same.
- Freezing: When water freezes, it changes from a liquid to a solid, but its chemical composition does not change.
- Cutting: When you cut a piece of paper, the paper’s size and shape change, but its chemical structure remains unchanged.
Chemical Changes
Chemical changes involve a change in the chemical composition of the substance. These changes result in the formation of new substances with different properties from the original substances. Chemical changes are usually irreversible. Examples of chemical changes include:
- Combustion: When wood burns, it reacts with oxygen to form carbon dioxide, water vapor, and ash. This process is a chemical change because new substances are formed.
- Oxidation: When iron rusts, it reacts with oxygen and water to form iron oxide, a new substance with different properties from iron.
- Decomposition: When hydrogen peroxide decomposes, it breaks down into water and oxygen gas, resulting in the formation of new substances.
Expressing a Physical Quantity
Physical quantities are always expressed in terms of two components:
- The numerical value: This represents the magnitude of the quantity.
- The unit: This represents the standard of measurement.
For example, the mass of a box might be stated as 6.5 kg. Here, 6.5 is the numerical value, and kilograms (kg) is the unit. The numerical value alone conveys no significant information; it must be accompanied by a unit to be meaningful.
Units of Measurement
A unit is a standard of reference used to measure physical quantities. To ensure consistency and accuracy in measurements, a standardized system of units is essential. The International System of Units (SI), also known as the Système International d’Unités, is the most widely used system of measurement in the world.
Fundamental and Derived Units
Fundamental units are the basic units of measurement that cannot be derived from any other unit. These include units for mass (kilogram), length (meter), time (second), temperature (Kelvin), electric current (ampere), luminous intensity (candela), and the amount of substance (mole).
Derived units are units that are derived from the fundamental units. Examples include:
- Newton (N): The unit of force, derived from kilograms, meters, and seconds.
- Joule (J): The unit of energy, derived from kilograms, meters, and seconds.
The Metric System
The metric system is a decimal-based system of measurement that was first introduced in France in 1791. It is based on powers of ten, making it easy to convert between different units. The metric system includes units such as the meter for length, the gram for mass, and the liter for volume.
In 1957, India adopted the metric system, and it has since become the standard system of measurement in most countries around the world.
SI Units of Physical Quantities
The SI system includes seven base units, which correspond to seven fundamental scientific quantities. These units are:
- Meter (m) for length
- Kilogram (kg) for mass
- Second (s) for time
- Ampere (A) for electric current
- Kelvin (K) for temperature
- Mole (mol) for the amount of substance
- Candela (cd) for luminous intensity
Although the Kelvin (K) is the SI unit for temperature, the Celsius scale (°C) is still commonly used in everyday life. The relationship between these two temperature scales is given by:
Temperature in Kelvin (K) = Temperature in Celsius (°C) + 273.15
Conclusion
The study of matter and its properties is fundamental to understanding the physical world. By exploring the various physical and chemical properties of matter, we gain insights into the behavior and characteristics of different materials. Whether we are measuring the density of a substance, observing a chemical reaction, or calculating the heat capacity of a material, the properties of matter provide a framework for analyzing and understanding the world around us.
As we continue to explore the properties of matter, we develop new technologies, create new materials, and deepen our understanding of the universe. The principles discussed in this exploration form the foundation of many scientific disciplines, from chemistry and physics to engineering and environmental science.
Informative Table Based on Properties of Matter
To help summarize the key concepts discussed in the exploration of the properties of matter, the following table presents an organized overview of the physical and chemical properties, along with associated examples and relevant details. This table serves as a quick reference guide for understanding the fundamental characteristics of matter and how they manifest in different substances.
Category | Property | Definition | Example | Additional Details |
---|---|---|---|---|
Physical Properties | Density | Mass per unit volume | Gold | $$ \text{Density} (\rho) = \frac{\text{Mass} (m)}{\text{Volume} (V)} $$ (example, g/cm³) |
Color | The appearance of matter based on light reflection/absorption | Blue sky, green leaves | Determined by the wavelengths of light | |
Hardness | Resistance to deformation or scratching | Diamond | Used in cutting tools and abrasives | |
Melting Point | Temperature at which a solid turns into a liquid | Ice (0°C) | Unique to each substance | |
Boiling Point | The temperature at which a liquid turns into a gas | Water (100°C) | Unique to each substance | |
Electrical Conductivity | Ability to conduct electric current | Copper, Silver | Metals have high conductivity | |
Malleability | Ability to be hammered into thin sheets | Gold | Property of metals | |
Ductility | Ability to be drawn into thin wires | Copper | Property of metals | |
Odor | Smell emitted by a substance | Vinegar (acetic acid), oranges (limonene) | Related to volatile compounds | |
Temperature | Measure of the average kinetic energy of particles | Water at 25°C | Measured in °C, K, or °F | |
Chemical Properties | Reactivity | Tendency to undergo chemical reactions | Potassium in water | Highly reactive substances |
Acidity | Ability to donate protons (H⁺) | Hydrochloric acid (HCl) | Reacts with bases to form salts | |
Basicity | Ability to accept protons | Ammonia (NH₃) | Reacts with acids | |
Flammability | Ability to burn in the presence of oxygen | Wood | Important in fire safety | |
Toxicity | Harmful effects on living organisms | Mercury, Lead | Can cause severe health issues | |
Heat of Combustion | Heat energy released during complete combustion | Methane (CH₄) | Important for fuels | |
Thermal Properties | Heat Capacity | Amount of heat required to raise temperature by 1°C | Water | Indicates thermal energy storage capacity |
Specific Heat | Heat capacity per unit mass | Sand, Water | Important in thermal management | |
Thermal Conductivity | Ability to conduct heat | Metals (Copper, Aluminum) | Metals are good conductors, insulators are not | |
Phase Changes | Transitions between solid, liquid, and gas | Ice melting, water boiling | Associated with heat absorption or release | |
Intensive Properties | Intensive Properties | Independent of the amount of matter present | Density, Temperature, Pressure | Inherent to the material |
Extensive Properties | Extensive Properties | Dependent on the amount of matter present | Mass, Volume, Total Energy | Varies with sample size |
Physical Changes | Physical Changes | Changes affecting state or appearance without altering the composition | Ice melting, paper cutting | Usually reversible |
Chemical Changes | Chemical Changes | Changes that alter the chemical composition, forming new substances | Combustion, rusting, decomposition | Usually irreversible |
Units and Measurement | SI Units | Standardized system for measuring physical quantities | Meter (m), Kilogram (kg), Second (s) | Ensures consistency in scientific measurements |
Fundamental Units | Basic units of measurement | Meter (m), Kilogram (kg), Second (s) | Cannot be derived from other units | |
Derived Units | Units derived from fundamental units | Newton (N), Joule (J) | Based on combinations of fundamental units | |
Metric System | Decimal-based system of measurement | Meter, Gram, Liter | Adopted worldwide, including India in 1957 |
This table encapsulates the essential properties of matter, providing a quick and informative overview for students, educators, and professionals alike.
SI Units of Physical Quantities Commonly Used in Chemistry
Below is a table of SI Units of Physical Quantities commonly used in chemistry:
Physical Quantity | SI Unit | Symbol | Definition | Example |
---|---|---|---|---|
Length | Meter | m | The duration of 9,192,631,770 periods of radiation corresponding to the transition between the two hyperfine levels of the ground state of the cesium-133 atom | The bond length between H and O in water is approximately 0.096 m |
Mass | Kilogram | kg | The mass of the International Prototype of the Kilogram | The mass of a water molecule is approximately $$ 2.99 \times 10^{-26} $$ kg |
Time | Second | s | The duration of 9,192,631,770 periods of radiation corresponding to the transition between the two hyperfine levels of the ground state of the cesium-133 atom | The time for a chemical reaction to complete might be 10 seconds |
Amount of Substance | Mole | mol | The amount of substance that contains as many entities as there are atoms in 0.012 kg of carbon-12 | 1 mole of water contains approximately $$ 6.022 \times 10^{23} $$ molecules |
Temperature | Kelvin | K | The fraction $$ \frac{1}{273.16} $$ of the thermodynamic temperature of the triple point of water | The standard room temperature is approximately 298 K |
Electric Current | Ampere | A | The current that, if maintained in two straight parallel conductors of infinite length, of negligible circular cross-section, and placed 1 meter apart in a vacuum, would produce a force equal to $$ 2 \times 10^{-7} $$ newtons per meter of length | The current in an electrolysis experiment might be 0.1 A |
Luminous Intensity | Candela | cd | The luminous intensity, in a given direction, of a source that emits monochromatic radiation of frequency $$ 540 \times 10^{12} $$ hertz and has a radiant intensity in that direction of $$ \frac{1}{683} $$ watt per steradian | The luminous intensity of a light bulb could be 100 cd |
Pressure | Pascal | Pa | One pascal is equivalent to one newton per square meter $$( \text{N/m}^2 )$$ | The atmospheric pressure at sea level is approximately 101,325 Pa |
Energy | Joule | J | One joule is the energy transferred when one newton of force is applied over a distance of one meter | The energy released by burning 1 gram of glucose is approximately 15.6 kJ |
Power | Watt | W | One watt is equal to one joule per second $$( \text{J/s} )$$ | The power output of a small light bulb might be 60 W |
Frequency | Hertz | Hz | One hertz is one cycle per second | The frequency of visible light is on the order of $$ 10^{14} $$ Hz |
Electric Charge | Coulomb | C | One coulomb is the charge transported by a steady current of one ampere in one second | The charge of an electron is approximately $$ -1.602 \times 10^{-19} $$ C |
Voltage | Volt | V | One volt is equal to one joule per coulomb $$( \text{J/C} )$$ | The voltage of a standard AA battery is 1.5 V |
Resistance | Ohm | Ω | One ohm is equal to one volt per ampere $$( \text{V/A} )$$ | The resistance of a typical household light bulb is about 240 Ω |
Capacitance | Farad | F | One farad is equal to one coulomb per volt $$( \text{C/V} )$$ | The capacitance of a small capacitor might be $$ 10^{-6} $$ F (1 µF) |
This table highlights the fundamental SI units used in chemistry, offering a concise reference for students and professionals.
Frequently Asked Questions (FAQs)
What is Matter?
Matter is defined as any substance that has mass and occupies space. Everything around us, including the air we breathe, the water we drink, and the objects we use daily, is made up of matter. Matter can exist in different states, primarily solid, liquid, and gas, and it consists of tiny particles known as atoms. These atoms are the fundamental building blocks of matter and combine to form molecules, which make up the various substances we encounter.
What are the Physical Properties of Matter?
Physical properties of matter are characteristics that can be observed or measured without altering the substance’s chemical composition. Examples include density, color, hardness, melting point, boiling point, and electrical conductivity. These properties help us identify and classify different types of matter. For instance, the density of a substance determines whether it will float or sink in water, and the melting point indicates the temperature at which a solid turns into a liquid.
What are the Chemical Properties of Matter?
Chemical properties are characteristics of a substance that become evident during a chemical reaction. These include reactivity, flammability, acidity, basicity, and toxicity. Unlike physical properties, chemical properties describe how a substance interacts with other substances to form new compounds. For example, the reactivity of potassium with water is a chemical property that results in the formation of potassium hydroxide and the release of hydrogen gas.
How do Physical and Chemical Changes Differ?
A physical change involves a change in the state or appearance of a substance without altering its chemical composition. Examples include melting, freezing, and cutting. A chemical change, on the other hand, results in the formation of one or more new substances with different chemical properties. This includes processes like combustion, oxidation, and decomposition. For example, burning wood is a chemical change that produces ash, carbon dioxide, and water.
What is Density, and How is it Calculated?
Density is defined as the mass of a substance per unit volume and is commonly expressed in grams per cubic centimeter (g/cm³). It is calculated using the formula:
$$
\text{Density} (\rho) = \frac{\text{Mass} (m)}{\text{Volume} (V)}
$$
For example, if a block of iron has a mass of 100 grams and a volume of 10 cm³, its density would be:
$$
\rho = \frac{100 \text{ g}}{10 \text{ cm}^3} = 10 \text{ g/cm}^3
$$
This high density is why iron sinks in water.
What is the Significance of the Melting and Boiling Points?
The melting point is the temperature at which a solid turns into a liquid, while the boiling point is the temperature at which a liquid turns into a gas. These points are critical for identifying substances and understanding their phase transitions. For example, the melting point of ice is 0°C, and its boiling point is 100°C at standard atmospheric pressure. These properties are crucial in processes like distillation and purification.
What is Specific Heat, and Why is it Important?
Specific heat is the amount of heat energy required to raise the temperature of one gram of a substance by one degree Celsius (°C) or one Kelvin (K). It is an important thermal property because it determines how a substance responds to heat. For example, water has a high specific heat, meaning it can absorb a lot of heat without a significant increase in temperature. This property makes water an excellent coolant in industrial processes and helps regulate temperature in living organisms.
What is Thermal Conductivity?
Thermal conductivity is a measure of a material’s ability to conduct heat. Substances with high thermal conductivity, such as metals like copper and aluminum, transfer heat quickly and are used in applications like cookware and heat exchangers. In contrast, materials with low thermal conductivity, such as wood and styrofoam, are used as insulators to prevent heat transfer.
What is Reactivity in Chemistry?
Reactivity refers to the tendency of a substance to engage in a chemical reaction with another substance. This property is crucial in predicting how substances will behave in different chemical environments. For example, sodium is highly reactive with water, producing sodium hydroxide and releasing hydrogen gas. Reactivity is influenced by factors like temperature, pressure, and the presence of catalysts.
What are Intensive and Extensive Properties?
Intensive properties are independent of the amount of matter present and include density, temperature, and pressure. These properties remain unchanged regardless of the size of the sample. Extensive properties, on the other hand, depend on the amount of matter and include mass, volume, and total energy. For instance, doubling the mass of a substance also doubles its volume, making mass an extensive property.
What is the Significance of the SI Unit System in Chemistry?
The International System of Units (SI) is the standard system of measurement used in science, including chemistry, to ensure consistency and accuracy in data. The SI system includes seven base units: meter (m) for length, kilogram (kg) for mass, second (s) for time, mole (mol) for the amount of substance, kelvin (K) for temperature, ampere (A) for electric current, and candela (cd) for luminous intensity. These units provide a universal language for scientists around the world.
What are Derived Units in Chemistry?
Derived units are units that are formed by combining the base SI units according to mathematical relationships. For example, velocity is measured in meters per second (m/s), which combines the SI units of length (meter) and time (second). Other examples include pressure measured in pascals (Pa), where 1 Pa equals 1 newton per square meter (N/m²), and energy measured in joules (J), where 1 J equals 1 newton-meter (N·m).
What is the Difference Between a Mole and a Molecule?
A mole is a unit of measurement in chemistry that represents 6.022 × 10²³ entities (Avogadro’s number) of a substance, whether they are atoms, molecules, ions, or electrons. A molecule, on the other hand, is a specific arrangement of atoms bonded together. For example, one mole of water (H₂O) contains 6.022 × 10²³ water molecules, each of which is composed of two hydrogen atoms and one oxygen atom.
How is Temperature Related to the Kinetic Energy of Particles?
Temperature is a measure of the average kinetic energy of the particles in a substance. Higher temperatures indicate that the particles are moving more rapidly. This relationship is crucial in understanding how temperature affects reaction rates and phase changes. For example, as the temperature of a gas increases, the speed of its molecules increases, leading to higher pressure if the gas is confined to a constant volume.
What is the Importance of the Kelvin Scale in Chemistry?
The Kelvin scale is the standard unit of temperature in the SI system. It is essential in scientific calculations because it starts at absolute zero (0 K), the point where all molecular motion ceases. Unlike the Celsius scale, which starts at the freezing point of water (0°C), the Kelvin scale allows for direct comparisons of thermal energy. For example, a temperature change of 1 K is equivalent to a change of 1°C, but Kelvin avoids negative temperatures and is more convenient for thermodynamic calculations.
Read More Articles
- Kingdom Animalia: Definition, Classification, And Characteristics
- The Deuteromycetes: A Comprehensive Study Of Imperfect Fungi
- Basidiomycetes: A Comprehensive Study Of Club Fungi
- Ascomycetes – A Detailed Exploration Of Characteristics, Reproduction, And Importance
- Phycomycetes: A Detailed Exploration Of The Lower Fungi
- Kingdom Fungi: A Comprehensive Exploration
- Protozoans: Structure, Classification, Characteristics, And Examples
- Slime Moulds: A Comprehensive Overview
- Euglenoid: The Unique Plant-Animal Hybrid
- Dinoflagellates: The Whirling Protists of the Oceans
- Chrysophytes: A Detailed Exploration Of Their Classification, Characteristics, & Significance
- Eubacteria: Structure, Characteristics, Classification, And Types
- Archaebacteria: A Glimpse Into Ancient Life Forms
- Kingdom Protista: A Diverse Group Of Single-Celled Eukaryotes
- The Diversity And Importance Of Bacteria: Archaebacteria And Eubacteria
- Kingdom Monera: The World Of Bacteria
- The Evolution Of Biological Classification Systems
- Understanding Taxonomy, Taxonomic Categories And Hierarchy
- The Diversity Of Life: Understanding Taxonomy, Classification, And Systematics