Measurement of Length is one of the most fundamental aspects of physics and engineering. The ability to measure length accurately and precisely is crucial in various scientific and industrial applications, from constructing buildings to designing intricate microchips. Length, a fundamental physical quantity, represents the distance between two points and is often considered the basis upon which other measurements are built. In this discussion, we will explore the principles of length measurement, delve into the various techniques and instruments used, and examine some real-world applications and examples.
Table of Contents
Principles of Length Measurement
The principle of length measurement is rooted in comparing an unknown length to a known standard. The standard unit of length in the International System of Units (SI) is the meter (m). Historically, the meter was defined based on a physical object, but modern definitions rely on constants of nature. Specifically, the meter is currently defined as the distance light travels in a vacuum in $$\frac{1}{299,792,458}$$ seconds. This definition links the meter directly to the speed of light, which is a fundamental constant of the universe.
The precision of length measurement is governed by several factors, including the accuracy of the measuring instrument, the environment in which the measurement is taken (such as temperature and pressure), and the skill of the person conducting the measurement.
For instance, measurements taken with a ruler may only be accurate to the nearest millimeter, whereas those taken with laser interferometers can be accurate to within fractions of a nanometer.
Techniques and Instruments for Measuring Length
- Rulers and Calipers:
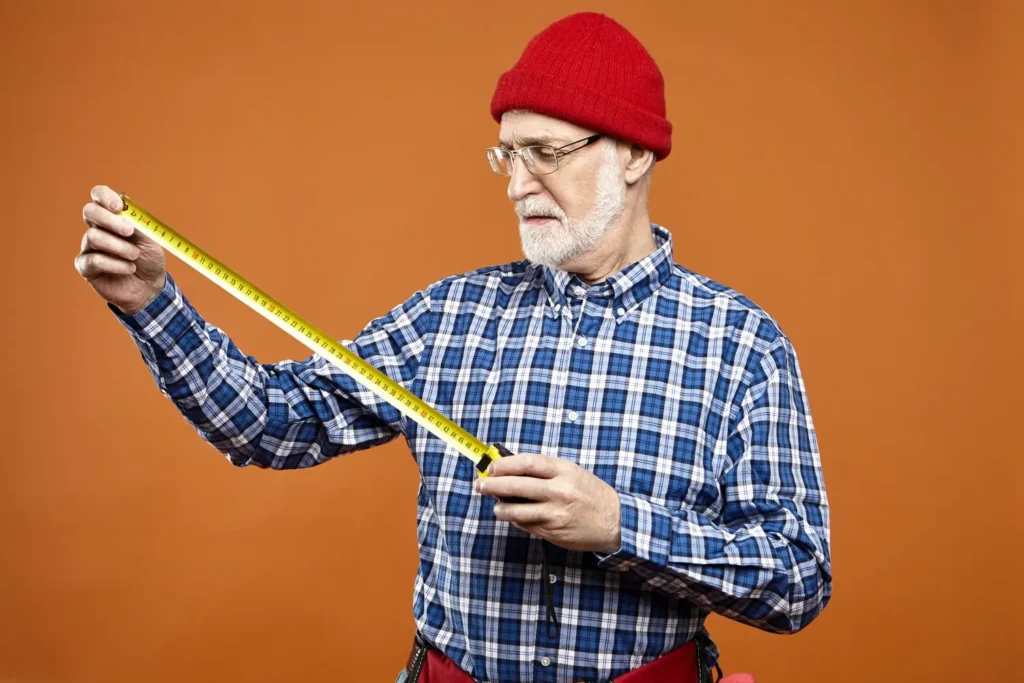
- Ruler: A ruler is a simple, yet effective tool for measuring length, particularly in the millimeter to meter range. It is typically marked in centimeters and millimeters. Although straightforward, the accuracy of a ruler depends on the precision of its markings and the user’s ability to align it correctly with the object being measured.
- Vernier Caliper: A vernier caliper is a more advanced tool that allows for more precise measurements than a standard ruler. It consists of a main scale and a sliding vernier scale that can measure length to the nearest 0.01 mm. The vernier caliper is especially useful for measuring the internal and external dimensions of objects as well as depths.
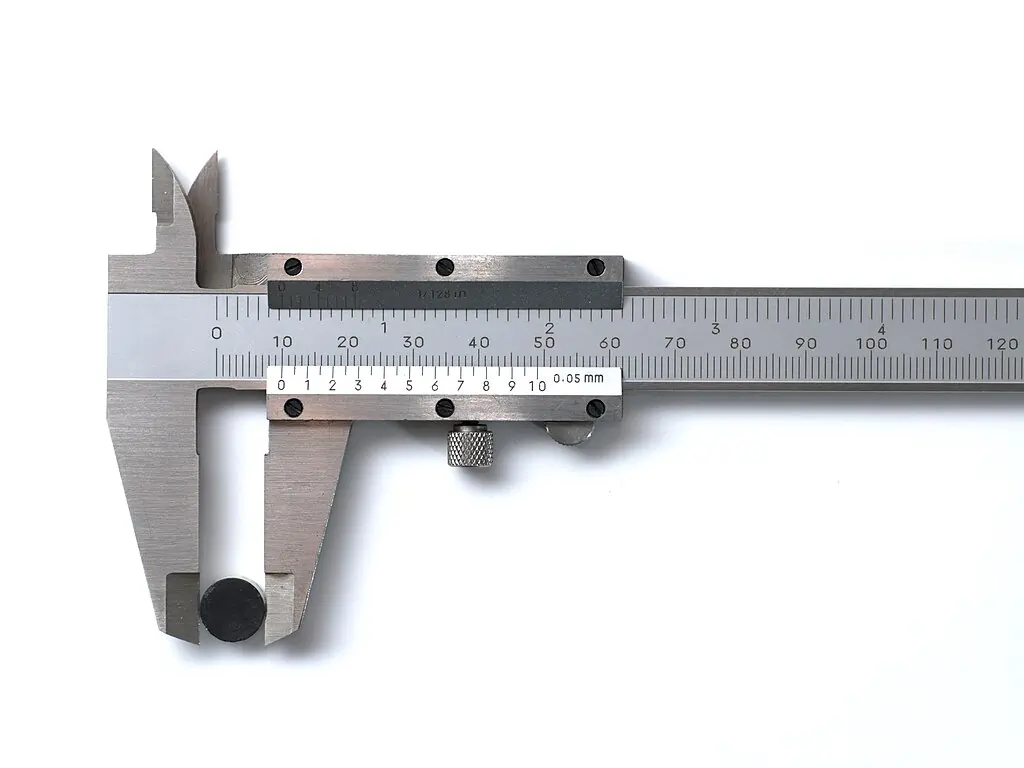
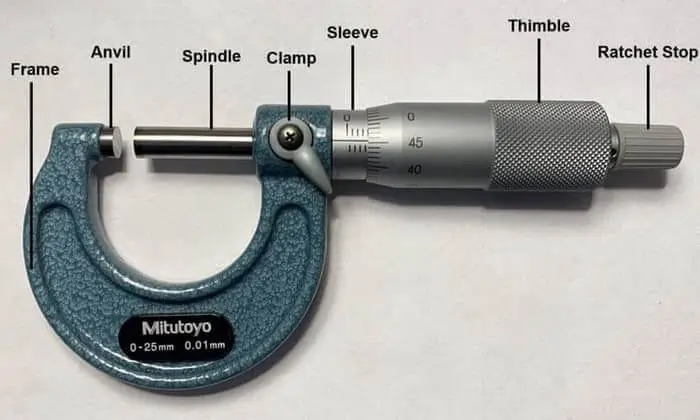
- Micrometer:
A micrometer is used to measure very small lengths with high precision. It typically has a spindle that moves in response to a thimble, which the user rotates. The precision of a micrometer can be up to 0.001 mm, making it suitable for applications requiring high accuracy, such as mechanical engineering. - Laser Interferometry:
Laser interferometry is a highly precise technique used for measuring very small distances or changes in distance. It works on the principle of interference, where two coherent light beams are combined, and the resulting interference pattern is analyzed. The accuracy of laser interferometry can reach sub-nanometer levels, making it invaluable in fields such as semiconductor manufacturing and optical engineering. $$ d = \frac{\lambda}{2} \times \frac{\Delta N}{\sin(\theta)} $$ where:
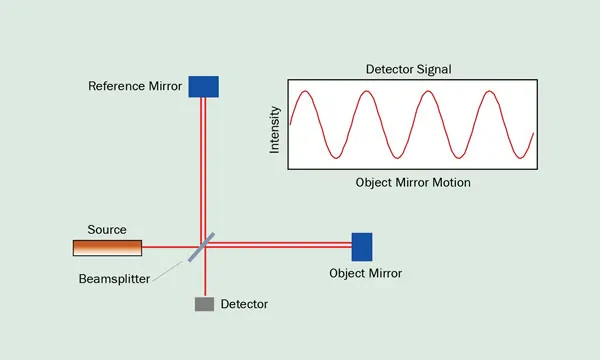
- $$d$$ is the distance being measured,
- $$\lambda$$ is the wavelength of the laser light,
- $$\Delta N$$ is the change in the number of fringes observed,
- $$\theta$$ is the angle of incidence.
- Surveying Instruments:
Surveying instruments like theodolites and total stations are used to measure large distances, often in the context of land surveying and construction. These instruments can measure angles and distances over kilometers with high precision.
Precision and Accuracy in Length Measurement
Precision refers to the consistency of repeated measurements, while accuracy describes how close a measurement is to the true value. In the context of length measurement, achieving both high precision and accuracy is essential. Errors can arise from various sources, including instrument calibration, environmental conditions, and human error.
For example, a micrometer screw gauge may provide highly precise measurements, but if the instrument is not calibrated correctly, the measurements may not be accurate. Similarly, temperature variations can cause materials to expand or contract, introducing errors in the measurement.
The formula for calculating measurement error is:
$$ \text{Error} = \left|\frac{\text{Measured Value} – \text{True Value}}{\text{True Value}}\right| \times 100\% $$
This formula helps in assessing the reliability of measurements and identifying potential sources of error.
Applications of Length Measurement
Length measurement plays a crucial role in various fields:
- Manufacturing:
In manufacturing, precise length measurements are critical for ensuring that components fit together correctly. This is especially important in the production of parts for machinery, electronics, and aerospace engineering, where even small deviations can lead to significant issues. - Construction:
Accurate length measurements are essential in construction to ensure that buildings, bridges, and other structures are built according to design specifications. Surveying instruments are often used to measure large distances and ensure the correct placement of structures. - Scientific Research:
In scientific research, particularly in physics and engineering, precise length measurements are required for experiments and the development of new technologies. For example, in optics, the measurement of wavelengths and the spacing between optical components must be extremely accurate to achieve the desired results.
Example: Measuring the Diameter of a Wire
Consider the task of measuring the diameter of a thin wire. This can be done using a micrometer. The wire is placed between the anvil and the spindle, and the thimble is rotated until the wire is securely held. The reading on the scale of the micrometer provides the diameter of the wire.
If the micrometer shows a reading of 0.25 mm, with an uncertainty of $$\pm 0.001$$ mm, the diameter of the wire can be expressed as:
$$ d = 0.25 \pm 0.001 \text{ mm} $$
This level of precision is often necessary in electrical engineering, where the diameter of wires affects their resistance and, consequently, the performance of electronic circuits.
Conclusion
The measurement of length is a foundational aspect of science and engineering. With advancements in technology, the precision and accuracy of length measurements have increased dramatically, enabling more complex and smaller-scale applications. From simple rulers to sophisticated laser interferometers, the tools and techniques available today allow us to measure lengths with incredible detail, ensuring the reliability and quality of products, research, and infrastructure in our modern world.
Frequently Asked Questions (FAQs)
What is the significance of length measurement in science and engineering?
Length measurement is a fundamental aspect of both science and engineering. In science, it is essential for quantifying the physical world, enabling researchers to describe phenomena with precision. For instance, in physics, the measurement of wavelengths of light is crucial for understanding electromagnetic radiation. In engineering, accurate length measurement ensures that components fit together correctly, which is critical for the functionality of machines, electronics, and structures. Precise length measurements also play a pivotal role in quality control processes, where even minor deviations can lead to significant performance issues or product failures. Thus, the ability to measure length accurately underpins the reliability, efficiency, and safety of scientific experiments and engineering projects.
How has the definition of the meter evolved?
The definition of the meter has undergone several changes since its inception, reflecting advancements in measurement technology and a deeper understanding of physical constants. Initially, the meter was defined in 1793 as one ten-millionth of the distance from the equator to the North Pole along a meridian through Paris. However, this was later replaced in 1889 by a standard meter bar made of platinum-iridium, which was stored at the International Bureau of Weights and Measures (BIPM) in France.
In 1960, with the advent of more precise measurement techniques, the meter was redefined in terms of the wavelength of light emitted by a krypton-86 atom. However, in 1983, the meter was redefined once again, this time based on the speed of light in a vacuum, which is a fundamental constant of nature. The current definition states that the meter is the distance light travels in a vacuum in $$\frac{1}{299,792,458}$$ seconds. This definition allows for extremely precise and reproducible measurements, crucial for advanced scientific research and high-tech industries.
What are the primary tools and instruments used for measuring length?
There are various tools and instruments available for measuring length, each suited to different levels of precision and applications:
- Ruler: A simple tool marked with units of length such as centimeters or inches, suitable for measurements within millimeters to meters.
- Vernier Caliper: An instrument that allows for more precise measurements, often used to measure internal and external dimensions as well as depths, with accuracy typically to 0.01 mm.
- Micrometer: A tool used for highly precise measurements, often down to 0.001 mm, making it ideal for applications in mechanical engineering where small tolerances are crucial.
- Laser Interferometer: A sophisticated instrument used for measuring very small distances or changes in distance with sub-nanometer precision. It is commonly used in fields like semiconductor manufacturing and optical engineering.
- Theodolite/Total Station: Surveying instruments used to measure large distances and angles in the context of land surveying and construction.
Each of these tools operates on different principles and offers varying levels of precision, allowing them to be used effectively in a wide range of scenarios.
How does temperature affect length measurement?
Temperature can significantly affect length measurement, particularly when dealing with materials that expand or contract with temperature changes. Most materials expand when heated and contract when cooled, which can introduce errors in measurements if not accounted for. This phenomenon is quantified by the coefficient of thermal expansion, which describes how much a material’s length changes per degree of temperature change.
For example, in precision engineering, where components must fit together with tight tolerances, even small temperature variations can lead to significant errors. This is why high-precision measurements are often conducted in controlled environments where the temperature is kept constant, or the measurement devices are calibrated to compensate for thermal expansion.
The formula to calculate the change in length due to temperature is:
$$ \Delta L = L_0 \alpha \Delta T $$
where:
- $$\Delta L$$ is the change in length,
- $$L_0$$ is the original length,
- $$\alpha$$ is the coefficient of thermal expansion,
- $$\Delta T$$ is the temperature change.
Understanding and compensating for thermal expansion is critical in applications such as aerospace, where components experience wide temperature ranges and must maintain their integrity and fit.
What is the difference between precision and accuracy in the context of length measurement?
Precision and accuracy are two important concepts in measurement, but they are not the same.
- Precision refers to the consistency of repeated measurements. If a measurement is precise, repeated measurements under the same conditions will yield very similar results. High precision is often represented by small standard deviations or low variability in measurements.
- Accuracy, on the other hand, refers to how close a measurement is to the true value. A measurement can be precise but not accurate if it consistently yields results that are not close to the actual value due to systematic errors. For example, if a ruler is incorrectly calibrated, it might consistently measure the length of an object as slightly longer than it is, resulting in precise but inaccurate measurements.
For an ideal measurement process, both high precision and high accuracy are desired. Techniques like calibration of instruments and repeated measurements are used to improve both aspects.
What is the role of laser interferometry in length measurement?
Laser interferometry is a technique that uses the interference of light waves to measure extremely small distances with high precision. It is based on the principle that when two coherent light beams are combined, they produce an interference pattern that can be analyzed to determine changes in distance.
The basic setup of a laser interferometer involves splitting a laser beam into two paths, with one beam reflecting off a fixed mirror and the other off a movable mirror. When the two beams are recombined, they produce an interference pattern that shifts as the movable mirror changes position. By analyzing this shift, it is possible to measure the distance moved by the mirror with incredible accuracy, often down to fractions of a nanometer.
Laser interferometry is used in a variety of high-precision applications, including the manufacturing of semiconductors, where precise control of layer thicknesses is essential, and in the calibration of other measurement instruments. Its ability to measure very small distances makes it invaluable in fields where traditional measurement tools cannot achieve the required precision.
How does one ensure the reliability of length measurements in industrial applications?
Ensuring the reliability of length measurements in industrial applications involves several key practices:
- Calibration: Regularly calibrating measuring instruments against known standards ensures that they provide accurate readings. Calibration involves comparing the instrument’s measurements with a standard reference and adjusting it as necessary.
- Environmental Control: Controlling environmental factors such as temperature, humidity, and vibration can prevent them from affecting measurements. For instance, precision measurements are often conducted in temperature-controlled environments to avoid thermal expansion effects.
- Use of Appropriate Tools: Selecting the right measuring tool for the task is crucial. For example, using a micrometer for small parts ensures greater precision than using a ruler.
- Training: Ensuring that personnel are properly trained in using measurement tools and techniques reduces the likelihood of human error. Proper training includes understanding how to align tools correctly, reading measurements accurately, and recognizing potential sources of error.
- Redundant Measurements: Taking multiple measurements and averaging them can reduce random errors. This practice helps to identify outliers and improve the overall accuracy of the measurement.
By implementing these practices, industries can maintain high standards of measurement reliability, which is essential for quality control, safety, and compliance with specifications.
What are some common sources of error in length measurement?
Several factors can introduce errors in length measurements:
- Instrumental Errors: These occur when the measuring instrument itself is flawed or improperly calibrated. For example, a ruler with worn markings or a micrometer with a misaligned spindle can lead to inaccurate measurements.
- Environmental Conditions: Temperature changes can cause materials to expand or contract, leading to errors. Humidity, pressure, and vibration can also affect the measurement process.
- Parallax Error: This occurs when the observer’s eye is not aligned perpendicularly to the scale being read. For instance, reading the markings on a ruler from an angle can result in an incorrect measurement.
- Human Error: Mistakes made by the person conducting the measurement, such as improper alignment of the instrument or misreading the scale, can introduce errors. Consistent training and practice are essential to minimize human error.
- Wear and Tear: Over time, measurement tools can wear out, especially those used frequently in industrial settings. Worn-out tools may no longer measure accurately, leading to errors.
- Zero Error: In instruments like vernier calipers and micrometers, zero error occurs when the instrument does not read zero when the measurement surfaces are in contact. This needs to be corrected by adjusting the reading or recalibrating the instrument.
Understanding these sources of error and implementing corrective measures can significantly improve the accuracy and precision of length measurements.
How do you measure the length of objects with irregular shapes?
Measuring the length of objects with irregular shapes can be challenging and often requires specialized techniques:
- Flexible Tape Measures: For measuring the perimeter or length of a curved or irregular object, flexible tape measures are often used. They can conform to the shape of the object, providing a more accurate measurement than rigid rulers.
- String Method: For very irregular shapes, a string can be used to follow the contour of the object. The string is then straightened and measured with a ruler or tape measure to determine the length.
- Calipers: For internal dimensions or small, irregular shapes, calipers can be used. The calipers can be adjusted to fit the shape, and the measurement can be taken from the scale.
- 3D Scanning: Advanced techniques like 3D scanning use lasers or structured light to capture the entire surface geometry of an object. The data collected can be processed to calculate the length, width, height, and other dimensions of even the most irregular shapes with high precision.
- Mathematical Approximations: In some cases, the shape can be approximated by a series of simple geometric shapes (examples, circles, triangles, rectangles), and the lengths of these segments can be summed to estimate the total length.
These methods enable accurate measurements even when dealing with complex or non-uniform objects, which is often necessary in fields like archaeology, medical imaging, and custom manufacturing.
What are the applications of length measurement in advanced technologies?
Length measurement is crucial in numerous advanced technologies, where precision and accuracy are paramount:
- Nanotechnology: In nanotechnology, precise measurements at the nanoscale are essential for manipulating materials and devices. Techniques like atomic force microscopy (AFM) rely on precise length measurements to image and manipulate atoms and molecules.
- Semiconductor Manufacturing: The production of semiconductors requires extremely precise length measurements to ensure that the components of integrated circuits are correctly sized and aligned. This precision is critical for the performance and reliability of electronic devices.
- Optical Engineering: In optical engineering, the precise measurement of wavelengths and the alignment of optical components are crucial for the development of lenses, lasers, and other optical devices. Laser interferometry is often used to achieve the required precision.
- Aerospace Engineering: In aerospace engineering, accurate length measurements are essential for the design and assembly of aircraft and spacecraft. Components must be manufactured to exact specifications to ensure the safety and performance of the vehicles.
- Medical Devices: In the medical field, length measurement is critical in the design and production of devices such as stents, catheters, and prosthetics, where precision ensures that the devices function correctly and fit the patient properly.
The importance of length measurement in these and other advanced technologies cannot be overstated, as it directly impacts the development, quality, and functionality of cutting-edge innovations.
Related Articles
- Range of Variation of Length in Measurement with Perfect Examples
- Understanding the Order of Magnitude with Perfect Examples
- The Advantages of SI Units with Proper Explanation
- Fundamental and Supplementary Units with Proper Explanation
- System of Units in Physics with Proper Explanation
- Unit of Time in Physics with Proper Explanation
- Unit of Length in Physics with Proper Explanation
- Unit of Mass in Physics: An In-Depth Exploration
- Characteristics of Standard Units in Physics with Proper Explanation
- Fundamental Units and Derived Units in Physics with Proper Explanation
- Units for Measurement in Physics with Proper Explanation
- The Crucial Role of Measurement in Physics with Proper Explanation
- The Nature of Physical Laws with Proper Explanation
- Fundamental Forces in Nature with Detailed Explanation
- Physics in Relation to Technology with Proper Explanation
- Physics in Relation to Society with Proper Explanation
- Physics in Relation to Science with Proper Explanation
- Physics: The Scope and Excitement of Physics
- Physics And Its Fundamentals With Good Explanations