The Laws of Chemical Combination are fundamental principles that underpin the field of chemistry. These laws describe how different types of matter interact, combine, and form new substances through chemical reactions. In our everyday lives, we observe various forms of matter reacting with one another—whether it’s the burning of fuel in engines, the rusting of iron, or even the cooking of food. These interactions, which often lead to the creation of entirely new substances, are governed by a set of well-defined rules known as the Laws of Chemical Combination.
Table of Contents
When a chemical reaction occurs, the properties, forms, and characteristics of the reactants change significantly, resulting in the formation of new compounds or elements. The Laws of Chemical Combination explain how these processes occur and ensure that chemical reactions follow predictable patterns.
Understanding the Laws of Chemical Combination
The Laws of Chemical Combination are a collection of principles that govern the interactions between different forms of matter. These laws dictate how substances combine to form new compounds and how the properties of these compounds are related to the properties of the original substances. There are five primary laws that make up the Laws of Chemical Combination:
- Law of Conservation of Mass
- Law of Definite Proportions
- Law of Multiple Proportions
- Gay-Lussac’s Law of Gaseous Volumes
- Avogadro’s Law
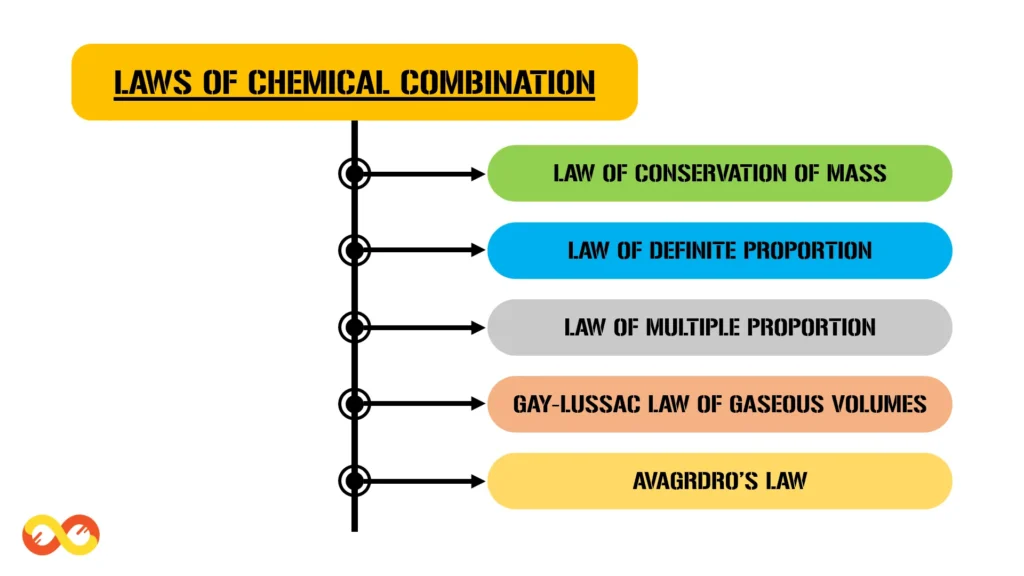
Each of these laws plays a crucial role in our understanding of chemical reactions and the formation of compounds. Let’s delve into each of these laws in detail, along with additional information and examples to illustrate their significance.
Law of Conservation of Mass
The Law of Conservation of Mass is one of the most fundamental principles in chemistry. It states that “Mass can neither be created nor destroyed in a chemical reaction,” but it can be transformed from one form to another. This law was first introduced by Mikhail Lomonosov in 1756 and later refined by Antoine Lavoisier in 1773 after extensive experimentation.
In simple terms, this law means that during any chemical or physical change within a closed system, the total mass of the reactants (the substances before the reaction) is exactly equal to the total mass of the products (the substances formed after the reaction). Since the system is closed, no reactants or products can escape, ensuring that the mass remains constant.
Example of the Law of Conservation of Mass
Consider the formation of a water molecule (H₂O) from hydrogen (H₂) and oxygen (O₂) molecules. The balanced chemical equation for this reaction is:
2H₂ + O₂ → 2H₂O
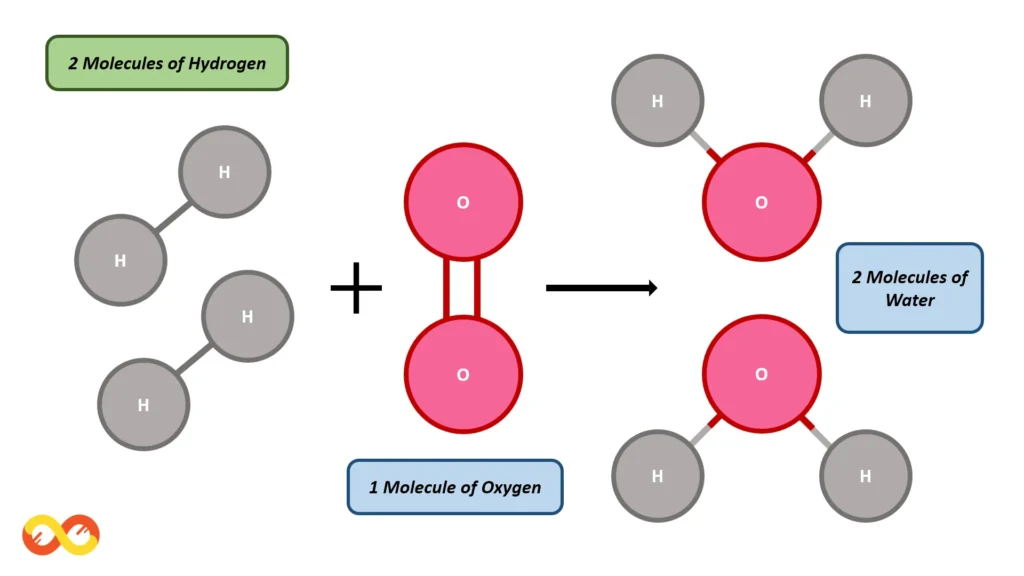
In this reaction, two molecules of hydrogen gas combine with one molecule of oxygen gas to form two molecules of water. To understand how mass is conserved, let’s break down the masses involved:
- The mass of one hydrogen atom (H) is approximately 1 atomic mass unit (amu), and since we have two molecules of H₂, the total mass of hydrogen in the reactants is 4 amu.
- The mass of one oxygen atom (O) is approximately 16 amu, and since we have one molecule of O₂, the total mass of oxygen in the reactants is 32 amu.
- The mass of two molecules of water (2H₂O) is 36 amu (since each water molecule contains two hydrogen atoms and one oxygen atom).
Thus, the total mass of the reactants (4 amu from hydrogen + 32 amu from oxygen = 36 amu) is equal to the total mass of the products (36 amu from water). This demonstrates the Law of Conservation of Mass in action, where the mass of the substances involved in a chemical reaction remains constant.
Additional Example: Combustion of Methane
Let’s consider the combustion of methane (CH₄), a common reaction that occurs when natural gas is burned:
CH₄ + 2O₂ → CO₂ + 2H₂O
In this reaction:
- The mass of one carbon atom (C) is 12 amu.
- The mass of four hydrogen atoms (H) is 4 amu.
- The mass of two oxygen molecules (2O₂) is 64 amu.
The total mass of the reactants (CH₄ + 2O₂) is 80 amu. The products are CO₂ (with a mass of 44 amu) and 2H₂O (with a mass of 36 amu), which also add up to 80 amu. This example further illustrates that the total mass remains unchanged during a chemical reaction.
Law of Definite Proportions
The Law of Definite Proportions, also known as the Law of Constant Composition, states that in any given chemical compound, the composition of the elements by mass always remains the same. This means that a specific chemical compound always contains its constituent elements in the same fixed ratio by mass, regardless of the sample size or source of the compound. This law was first proposed by Joseph Louis Proust in the late 18th century.
For instance, water (H₂O) always contains hydrogen and oxygen in a mass ratio of 1:8. This means that for every 1 gram of hydrogen, there are 8 grams of oxygen in the compound. This ratio remains constant whether you are examining a drop of water or an entire ocean.
Example of the Law of Definite Proportions
Consider the compound sodium chloride (NaCl), commonly known as table salt. Sodium chloride always contains sodium (Na) and chlorine (Cl) in a 1:1 molar ratio. By mass, sodium makes up approximately 39.3% of the compound, while chlorine makes up about 60.7%. This ratio remains the same regardless of the amount of sodium chloride you have.
Consider the different molecules of Oxides of Nitrogen for examples of the law of definite proportions. In the above example of oxides of Nitrogen if we take any amount of Oxygen and Nitrogen in the sample then the ratio of nitrogen to oxygen in the formed NO will always be 1:1. Similarly, the ratio of nitrogen to oxygen for NO2, N2O, and N2O2 will be 1:2, 2:1 and 2:2 respectively. The law of definite proportions guarantees this.
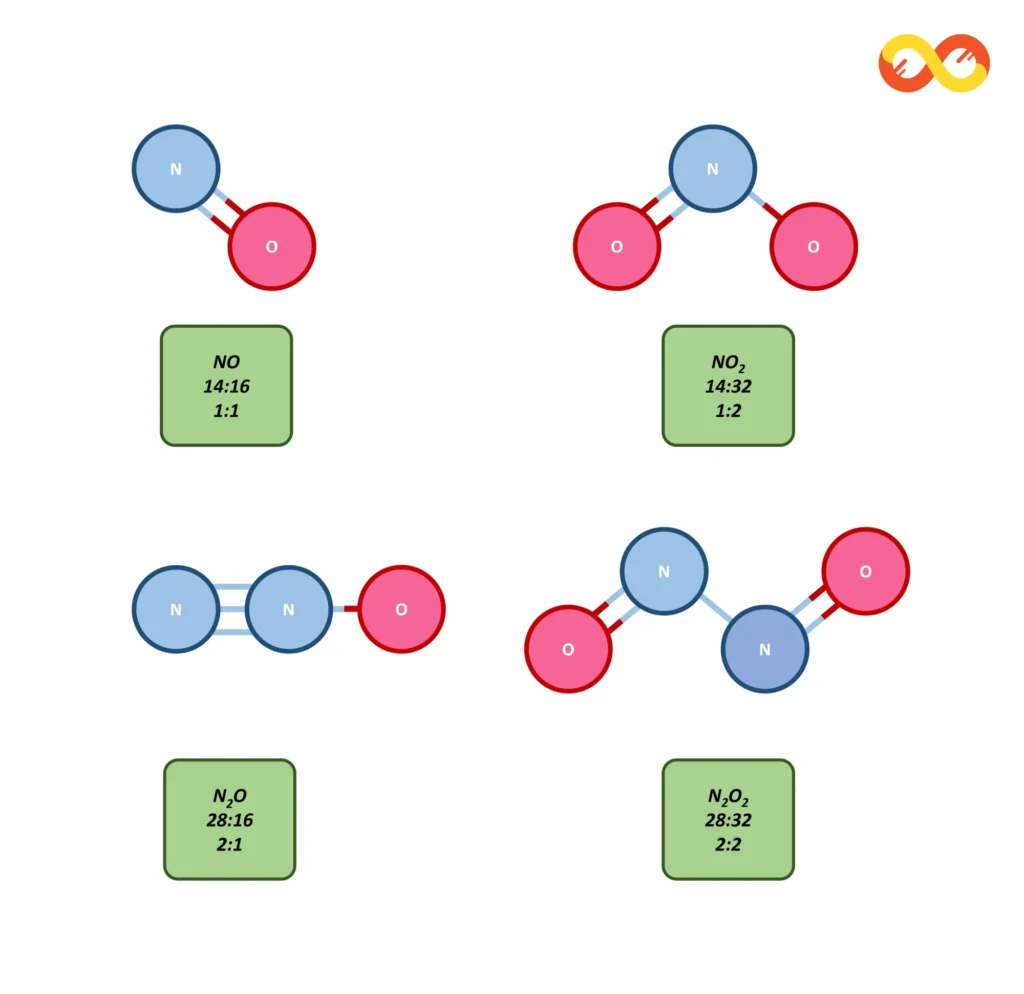
Additional Example: Carbon Dioxide
Another example is carbon dioxide (CO₂), a compound composed of carbon (C) and oxygen (O). In CO₂, the ratio of the mass of carbon to oxygen is always 12:32 or 3:8. This ratio remains constant whether you’re examining a small sample of carbon dioxide or a large one.
The Law of Definite Proportions is crucial for identifying different chemical compounds. For instance, carbon monoxide (CO) and carbon dioxide (CO₂) both contain carbon and oxygen, but in different ratios. The mass ratio of carbon to oxygen in CO is 12:16 or 3:4, whereas in CO₂, as mentioned earlier, it is 3:8. This difference in ratios allows us to distinguish between the two compounds.
Law of Multiple Proportions
The Law of Multiple Proportions is another fundamental principle of chemistry, first proposed by John Dalton in 1804. This law states that when two elements combine to form more than one compound, the masses of one element that combine with a fixed mass of the other element are in a ratio of small whole numbers.
In simpler terms, if two elements can form multiple compounds, the ratio of the masses of one element that combines with a fixed mass of the other element will always be a small, whole number.
Example of the Law of Multiple Proportions
Let’s examine the compounds formed by carbon and oxygen:
- Carbon monoxide (CO)
- Carbon dioxide (CO₂)
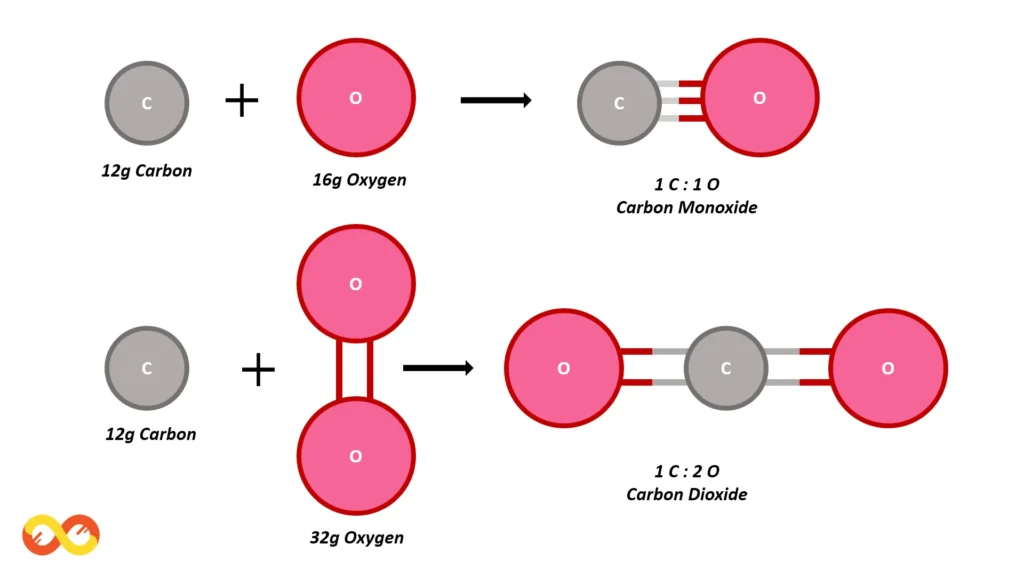
In carbon monoxide (CO), 12 grams of carbon combine with 16 grams of oxygen. In carbon dioxide (CO₂), 12 grams of carbon combine with 32 grams of oxygen. The ratio of the mass of oxygen in the two compounds is 32:16, which simplifies to 2:1, a simple whole-number ratio.
Additional Example: Nitrogen Oxides
The oxides of nitrogen provide another excellent example of the Law of Multiple Proportions. Nitrogen forms several oxides, including nitrous oxide (N₂O), nitric oxide (NO), and nitrogen dioxide (NO₂).
- In nitrous oxide (N₂O), 14 grams of nitrogen combine with 8 grams of oxygen.
- In nitric oxide (NO), 14 grams of nitrogen combine with 16 grams of oxygen.
- In nitrogen dioxide (NO₂), 14 grams of nitrogen combine with 32 grams of oxygen.
The ratios of the masses of oxygen that combine with a fixed mass of nitrogen (14 grams) are 8:16:32, which simplifies to 1:2:4. Again, we see that the masses of oxygen in these different compounds are in simple whole number ratios, illustrating the Law of Multiple Proportions.
Gay-Lussac’s Law of Gaseous Volumes
Gay-Lussac’s Law of Gaseous Volumes, formulated by Joseph Louis Gay-Lussac in 1808, states that “When gases are produced or combined in a chemical reaction, they do so in simple volume ratios, provided that all the gases are at the same temperature and pressure.” This law is essentially an extension of the Law of Definite Proportions, but it applies specifically to gases and deals with volumes instead of masses.
Example of Gay-Lussac’s Law of Gaseous Volumes
Consider the reaction between hydrogen and oxygen to form water vapor:
2H₂ (g) + O₂ (g) → 2H₂O (g)
In this reaction, two volumes of hydrogen gas combine with one volume of oxygen gas to produce two volumes of water vapor. The volume ratio of hydrogen to oxygen to water is 2:1:2, which is a simple whole-number ratio.
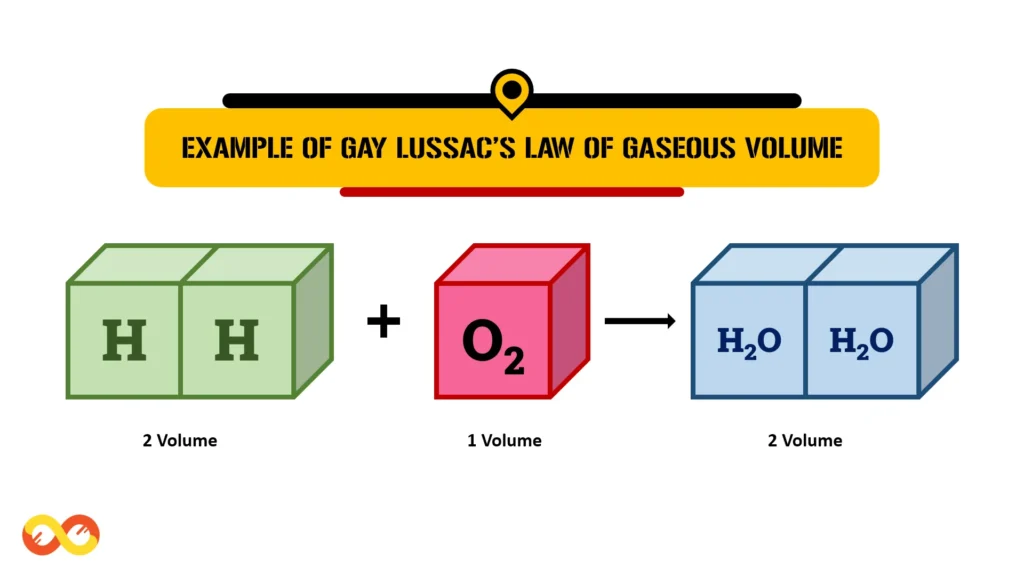
Additional Example: Formation of Ammonia
Another example is the formation of ammonia (NH₃) from nitrogen and hydrogen gases:
N₂ (g) + 3H₂ (g) → 2NH₃ (g)
Here, one volume of nitrogen gas reacts with three volumes of hydrogen gas to produce two volumes of ammonia gas. The volume ratio is 1:3:2, again a simple whole number ratio, in accordance with Gay-Lussac’s Law.
This law is particularly important in the study of gas reactions, as it allows chemists to predict the volumes of gases involved in a reaction, provided the temperature and pressure remain constant.
Avogadro’s Law
Avogadro’s Law is another cornerstone of modern chemistry. Proposed by Amedeo Avogadro in 1811, this law states that “Equal volumes of all gases, at the same temperature and pressure, contain the same number of molecules.” In other words, the volume of a gas is directly proportional to the number of moles of the gas when the temperature and pressure are kept constant.
Avogadro’s Law is mathematically expressed as:
V ∝ n
Where V is the volume of the gas, and n is the number of moles of the gas. This means that if you have two containers of gas at the same temperature and pressure, and they have the same volume, they will also contain the same number of molecules, regardless of the type of gas.
Example of Avogadro’s Law
Example 1: Consider two gases: hydrogen (H₂) and oxygen (O₂). If you have one liter of hydrogen gas and one liter of oxygen gas at the same temperature and pressure, both containers will have the same number of molecules, even though hydrogen is much lighter than oxygen.
Example 2: Equal volumes of different gases, under the same conditions of temperature and pressure, contain an identical number of molecules. In the given example, one volume of chlorine gas (Cl₂) reacts with one volume of hydrogen gas (H₂) to produce two volumes of hydrogen chloride (HCl).
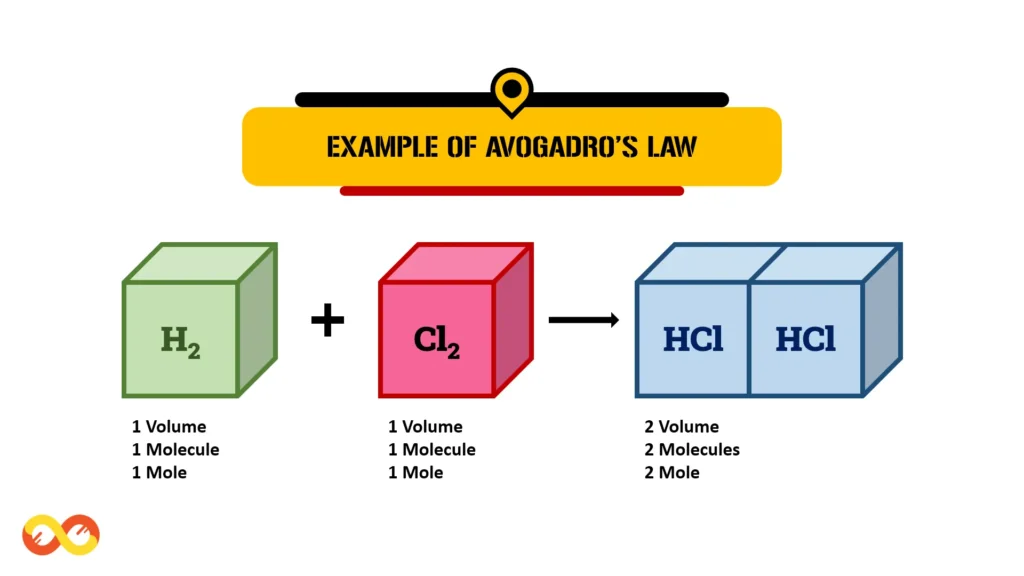
Here, the concept of a mole serves as a fundamental unit in chemistry, representing the quantity of a substance. One mole of any substance contains exactly 6.02214076 × 10²³ particles, be it atoms, molecules, or ions.
Additional Example: Comparing Different Gases
Let’s compare chlorine (Cl₂) and nitrogen (N₂) gases. If both are at the same temperature and pressure and occupy the same volume, they will contain the same number of molecules. This is true even though chlorine is a much heavier gas than nitrogen.
This concept is critical in understanding gas behavior and is foundational in the study of molar volume and the Ideal Gas Law. It also leads to the understanding of the mole, a standard unit of measurement in chemistry, where one mole of any gas at standard temperature and pressure (STP) occupies 22.4 liters.
Informative Table Based on Laws of Chemical Combination
The Laws of Chemical Combination form the foundation of our understanding of chemical reactions and the behavior of matter during these reactions. These laws explain how different elements combine to form compounds and how the properties of these compounds are governed by fixed ratios and consistent patterns. Below is a detailed table summarizing the key aspects of each of these fundamental laws, including their definitions, historical background, and examples to illustrate their significance in chemistry.
Law | Definition | Proposed By | Year | Key Points | Example |
---|---|---|---|---|---|
Law of Conservation of Mass | Mass can neither be created nor destroyed in a chemical reaction but can change form. | Mikhail Lomonosov | 1756 | – Total mass of reactants equals total mass of products. – Mass remains constant in a closed system. | Formation of water (H₂O): 2H₂ + O₂ → 2H₂O; Mass of reactants (36 amu) equals mass of products (36 amu). |
Law of Definite Proportions | In any given compound, the composition of elements by mass is always the same. | Joseph Louis Proust | Late 18th century | – Fixed mass ratio in chemical compounds. – Used to distinguish different compounds. | Water (H₂O): Always contains hydrogen and oxygen in a 1:8 mass ratio. |
Law of Multiple Proportions | If two elements form more than one compound, the ratio of masses of one element that combines with a fixed mass of the other element is always a small whole number. | John Dalton | 1804 | – The Ratio of masses is a simple whole number. – Helps in identifying multiple compounds formed by the same elements. | Carbon oxides (CO and CO₂): The Ratio of oxygen in CO₂ to CO is 2:1 (32g/16g). |
Gay-Lussac’s Law of Gaseous Volumes | When gases react, they do so in simple volume ratios, provided they are at the same temperature and pressure. | Joseph Louis Gay-Lussac | 1808 | – Volume ratios in gas reactions are simple whole numbers. – Applies specifically to gases. | Formation of water vapor (H₂O): 2 volumes of H₂ combine with 1 volume of O₂ to form 2 volumes of H₂O. |
Avogadro’s Law | Equal volumes of all gases, at the same temperature and pressure, contain the same number of molecules. | Amedeo Avogadro | 1811 | – The Volume of a gas is directly proportional to the number of moles. – Important for understanding gas behavior and molar volume. | Equal volumes of H₂ and O₂: At the same temperature and pressure, both have the same number of molecules, despite their different masses. |
This table serves as a comprehensive overview of the fundamental Laws of Chemical Combination, illustrating their key concepts, historical significance, and practical applications in the study of chemistry.
Conclusion
The Laws of Chemical Combination are fundamental principles that provide a comprehensive understanding of how chemical reactions occur and how different forms of matter combine to create new substances. These laws—Law of Conservation of Mass, Law of Definite Proportions, Law of Multiple Proportions, Gay-Lussac’s Law of Gaseous Volumes, and Avogadro’s Law—form the bedrock of chemical theory and practice.
Understanding these laws not only helps chemists predict the outcomes of reactions but also allows for the accurate measurement and manipulation of chemical substances. Whether you’re mixing chemicals in a lab, studying the composition of the atmosphere, or developing new materials, these laws guide your understanding of the molecular world. By adhering to these principles, chemists can unravel the complexities of matter and continue to make groundbreaking discoveries in the field of chemistry.
Related Articles
- Understanding Measurement Uncertainty in Chemistry with Perfect Explanation
- Properties of Matter: An In-Depth Exploration
- Understanding Matter: States, Properties, and Beyond
- The Importance of Chemistry in Everyday Life
Frequently Asked Questions (FAQs) about the Laws of Chemical Combination
What are the Laws of Chemical Combination?
The Laws of Chemical Combination are a set of fundamental principles that govern how different elements combine to form compounds. These laws include the Law of Conservation of Mass, the Law of Definite Proportions, the Law of Multiple Proportions, Gay-Lussac’s Law of Gaseous Volumes, and Avogadro’s Law. They help chemists understand and predict the outcomes of chemical reactions.
Why is the Law of Conservation of Mass important in chemistry?
The Law of Conservation of Mass is crucial because it establishes that during any chemical reaction, the total mass of the reactants equals the total mass of the products. This principle is foundational for stoichiometry, which involves calculating the relative quantities of reactants and products in chemical reactions.
Who first proposed the Law of Conservation of Mass?
The Law of Conservation of Mass was first proposed by Mikhail Lomonosov in 1756 and was later refined and popularized by Antoine Lavoisier in 1773. Lavoisier’s experiments provided strong evidence for this law, establishing it as a cornerstone of modern chemistry.
Can the Law of Conservation of Mass be violated?
No, the Law of Conservation of Mass cannot be violated in ordinary chemical reactions. However, in nuclear reactions, mass can be converted into energy according to Einstein’s equation (E=mc²), but this is a different context from traditional chemical reactions.
What does the Law of Definite Proportions state?
The Law of Definite Proportions states that a given chemical compound always contains the same proportion of elements by mass. For example, water (H₂O) always consists of hydrogen and oxygen in a mass ratio of 1:8.
How does the Law of Definite Proportions help in identifying compounds?
The Law of Definite Proportions helps in distinguishing between different compounds that may contain the same elements. For example, water (H₂O) and hydrogen peroxide (H₂O₂) both contain hydrogen and oxygen, but in different mass ratios, which helps in their identification.
Who proposed the Law of Definite Proportions?
The Law of Definite Proportions was proposed by Joseph Louis Proust in the late 18th century. His experiments demonstrated that the composition of a chemical compound is always consistent, regardless of its source or method of preparation.
What is the significance of the Law of Multiple Proportions?
The Law of Multiple Proportions is significant because it explains how two elements can combine to form more than one compound with different properties. It shows that the masses of one element that combine with a fixed mass of another element are in a simple whole-number ratio.
Can you give an example of the Law of Multiple Proportions?
Yes, carbon and oxygen can combine to form two different compounds: carbon monoxide (CO) and carbon dioxide (CO₂). In these compounds, the ratio of the mass of oxygen that combines with a fixed mass of carbon is 2:1, illustrating the Law of Multiple Proportions.
Who discovered the Law of Multiple Proportions?
The Law of Multiple Proportions was discovered by John Dalton in 1804. Dalton’s work laid the foundation for his atomic theory, which proposed that matter is composed of indivisible atoms.
What does Gay-Lussac’s Law of Gaseous Volumes state?
Gay-Lussac’s Law of Gaseous Volumes states that when gases combine or are produced in a chemical reaction, they do so in a simple ratio by volume, provided all gases are at the same temperature and pressure.
How is Gay-Lussac’s Law of Gaseous Volumes applied in chemical reactions?
This law is applied in gas reactions to predict the volumes of gases involved. For example, in the reaction of hydrogen with oxygen to form water vapor, two volumes of hydrogen combine with one volume of oxygen to produce two volumes of water vapor.
Who formulated Gay-Lussac’s Law of Gaseous Volumes?
Gay-Lussac’s Law of Gaseous Volumes was formulated by Joseph Louis Gay-Lussac in 1808. His experiments with gases provided important insights into the relationships between volume, temperature, and pressure.
What is Avogadro’s Law?
Avogadro’s Law states that equal volumes of all gases, at the same temperature and pressure, contain the same number of molecules. This law is fundamental in understanding gas behavior and the concept of the mole.
Why is Avogadro’s Law important in chemistry?
Avogadro’s Law is important because it provides a direct relationship between the volume of a gas and the number of molecules it contains. This law is essential for calculating molar volumes and is a key component of the Ideal Gas Law.
Who proposed Avogadro’s Law?
Avogadro’s Law was proposed by Amedeo Avogadro in 1811. His work laid the groundwork for the modern understanding of gases and the development of the Ideal Gas Law.
How is the mole concept related to Avogadro’s Law?
The mole concept is directly related to Avogadro’s Law. One mole of any substance contains 6.022 × 10²³ particles (Avogadro’s number). According to Avogadro’s Law, one mole of any gas at standard temperature and pressure (STP) occupies 22.4 liters.
What is the Ideal Gas Law, and how is it connected to the Laws of Chemical Combination?
The Ideal Gas Law is an equation of state for a hypothetical ideal gas. It is represented as PV = nRT, where P is pressure, V is volume, n is the number of moles, R is the gas constant, and T is temperature. This law connects the Laws of Chemical Combination by incorporating Avogadro’s Law, Boyle’s Law, and Charles’s Law into a single equation.
How do the Laws of Chemical Combination apply to real-world scenarios?
The Laws of Chemical Combination apply to numerous real-world scenarios, from industrial chemical manufacturing to environmental science. For example, understanding the Law of Conservation of Mass is critical in designing chemical processes that minimize waste, while Gay-Lussac’s Law is important in understanding how gases behave under different conditions, such as in the atmosphere.
Are there any limitations to the Laws of Chemical Combination?
While the Laws of Chemical Combination are generally accurate, they have limitations when dealing with non-stoichiometric compounds, polymers, and nuclear reactions. For instance, Dalton’s Law of Multiple Proportions does not apply to non-stoichiometric compounds where the ratio of elements does not follow simple whole numbers. Similarly, the Law of Conservation of Mass is not applicable in nuclear reactions where mass can be converted into energy.
How does the Law of Conservation of Mass apply to closed and open systems in chemical reactions?
The Law of Conservation of Mass is strictly applicable in a closed system, where no matter can enter or leave during a chemical reaction. In a closed system, the total mass of the reactants before the reaction is exactly equal to the total mass of the products after the reaction. This is because there is no exchange of matter with the surroundings, ensuring that all atoms are accounted for, and their total mass remains unchanged.
In an open system, however, matter can be exchanged with the surroundings. For instance, gases may escape, or additional substances might be added during the reaction. In such cases, while the Law of Conservation of Mass still holds within the system, it may appear violated if only a portion of the system is observed. For example, in the combustion of fuel in an open container, the mass of the fuel decreases as gases like carbon dioxide (CO₂) and water vapor (H₂O) escape into the atmosphere. If these escaping gases are not accounted for, it might seem that mass is lost. However, when considering the entire system, including the gases, the total mass remains conserved.
How does the Law of Definite Proportions explain the difference between pure substances and mixtures?
The Law of Definite Proportions distinguishes between pure substances and mixtures based on the consistency of their chemical composition. In a pure substance, such as a compound, the ratio of elements by mass is always fixed and consistent, regardless of the sample size or source. For example, in any sample of pure water (H₂O), the mass ratio of hydrogen to oxygen will always be 1:8.
In contrast, mixtures are composed of two or more substances that are physically combined but not chemically bonded. The composition of a mixture can vary, and the proportions of the individual components are not fixed. For example, air is a mixture of gases like nitrogen (N₂), oxygen (O₂), carbon dioxide (CO₂), and others. The proportion of these gases can vary depending on location, altitude, and environmental conditions.
The Law of Definite Proportions does not apply to mixtures because their components can be present in any proportion. This law is specific to chemical compounds, where the elements are chemically bonded in a fixed ratio, forming a substance with consistent properties.
How did Dalton’s atomic theory relate to the Law of Multiple Proportions?
John Dalton’s atomic theory, proposed in the early 19th century, was closely related to the Law of Multiple Proportions. Dalton’s atomic theory postulated that all matter is composed of indivisible atoms and that these atoms combine in simple whole-number ratios to form compounds. This theory provided a theoretical framework that explained the observations described by the Law of Multiple Proportions.
According to this law, when two elements combine to form more than one compound, the masses of one element that combine with a fixed mass of the other element are in a simple whole-number ratio. Dalton explained this by suggesting that different compounds are formed when atoms of the elements combine in different whole-number ratios.
For example, carbon and oxygen can combine to form carbon monoxide (CO) and carbon dioxide (CO₂). In CO, one atom of carbon combines with one atom of oxygen. In CO₂, one atom of carbon combines with two atoms of oxygen. The ratio of the masses of oxygen that combine with a fixed mass of carbon in these two compounds is 2:1, reflecting the different atomic ratios.
Dalton’s atomic theory not only supported the Law of Multiple Proportions but also laid the groundwork for modern chemistry by introducing the concept of atoms and the way they combine to form compounds.
What role does Gay-Lussac’s Law of Gaseous Volumes play in understanding stoichiometry in gas reactions?
Gay-Lussac’s Law of Gaseous Volumes is vital in understanding stoichiometry in gas reactions because it establishes that when gases react, they do so in simple, whole-number volume ratios under the same conditions of temperature and pressure. This law is particularly useful in predicting the volumes of reactant and product gases involved in a chemical reaction.
In stoichiometry, which is the calculation of the relative quantities of reactants and products in chemical reactions, Gay-Lussac’s Law simplifies the process by allowing chemists to work directly with volumes rather than having to convert to mass. For example, in the reaction of hydrogen with oxygen to form water vapor:
2H₂ (g) + O₂ (g) → 2H₂O (g)
Gay-Lussac’s Law tells us that 2 volumes of hydrogen react with 1 volume of oxygen to produce 2 volumes of water vapor, provided that the gases are at the same temperature and pressure. This volume relationship is consistent regardless of the actual volume used, making it easier to predict the outcomes of reactions involving gases.
This law is particularly important in industrial applications where large volumes of gases are involved, such as in the production of ammonia (NH₃) through the Haber process or in the combustion of hydrocarbons.
How does Avogadro’s Law explain the relationship between the volume of a gas and the number of moles?
Avogadro’s Law states that under the same conditions of temperature and pressure, equal volumes of all gases contain the same number of molecules. This law implies that the volume of a gas is directly proportional to the number of moles of the gas present.
Mathematically, Avogadro’s Law can be expressed as:
V ∝ n (when T and P are constant),
where V is the volume of the gas, and n is the number of moles.
This relationship is crucial in determining the amount of gas involved in a reaction or present in a container. For instance, if you double the number of moles of a gas while keeping the temperature and pressure constant, the volume will also double. This principle is fundamental in gas stoichiometry, where the volume of gas can be calculated using the number of moles and vice versa.
Avogadro’s Law also underpins the concept of molar volume, which is the volume occupied by one mole of a gas at standard temperature and pressure (STP). At STP, one mole of any ideal gas occupies 22.4 liters. This concept is widely used in calculations involving gases in chemistry.
What is the significance of Avogadro’s number, and how is it related to Avogadro’s Law?
Avogadro’s number, which is 6.022 × 10²³, is the number of atoms, molecules, or other particles in one mole of a substance. This constant is fundamental in chemistry because it provides a bridge between the microscopic world of atoms and molecules and the macroscopic world that we can measure and observe.
Avogadro’s number is directly related to Avogadro’s Law, which states that equal volumes of all gases, at the same temperature and pressure, contain the same number of molecules. By establishing that one mole of any substance contains 6.022 × 10²³ particles, Avogadro’s number allows chemists to calculate the number of atoms, molecules, or ions in a given amount of substance.
For example, if you have 22.4 liters of oxygen gas at STP, you can confidently state that this volume contains 6.022 × 10²³ molecules of oxygen because one mole of gas at STP occupies 22.4 liters. This relationship is crucial for understanding and performing calculations in chemical reactions, especially when dealing with gases.
How did the discovery of the Laws of Chemical Combination contribute to the development of modern chemistry?
The discovery of the Laws of Chemical Combination was pivotal in the development of modern chemistry. These laws provided a systematic understanding of how elements combine to form compounds and established the principles of chemical reactions. The key contributions include:
- Quantitative Analysis: The Law of Conservation of Mass introduced the concept that mass is conserved in chemical reactions, leading to the development of quantitative chemistry, where precise measurements became central to understanding chemical processes.
- Atomic Theory: The Law of Multiple Proportions supported John Dalton’s atomic theory, which proposed that matter is composed of indivisible atoms. This theory laid the groundwork for modern atomic models and the concept of molecular structures.
- Chemical Formulas: The Law of Definite Proportions helped chemists define chemical formulas for compounds, ensuring that the same compound always has the same proportion of elements, which is crucial for the study of stoichiometry and chemical synthesis.
- Gas Laws and Molecular Theory: Gay-Lussac’s Law and Avogadro’s Law contributed to the understanding of gases and the development of the Ideal Gas Law, a cornerstone of physical chemistry.
Overall, these laws transformed chemistry
from a qualitative to a quantitative science, allowing for the precise calculation and prediction of chemical reactions and the development of new materials and technologies.
How are the Laws of Chemical Combination applied in industrial chemistry?
In industrial chemistry, the Laws of Chemical Combination are applied to optimize chemical processes, improve yield, and ensure the efficient use of resources. These laws are foundational in various industries, such as:
- Chemical Manufacturing: The Law of Conservation of Mass is crucial in designing reactors and processes that minimize waste. For example, in the production of sulfuric acid (H₂SO₄) via the Contact Process, careful accounting of the mass of reactants and products ensures high efficiency and minimal by-products.
- Pharmaceuticals: The Law of Definite Proportions ensures that pharmaceutical compounds are synthesized with the correct chemical composition, guaranteeing the safety and efficacy of drugs. For instance, the precise ratio of elements in aspirin (C₉H₈O₄) is critical for its therapeutic effect.
- Petrochemicals: In the petrochemical industry, Gay-Lussac’s Law is applied in processes involving gases, such as cracking and reforming, where understanding the volumes of gases involved is crucial for optimizing reaction conditions and maximizing output.
- Agriculture: The Haber process for producing ammonia (NH₃) relies on Avogadro’s Law to calculate the volumes of nitrogen and hydrogen gases required, ensuring the efficient production of fertilizers.
These laws ensure that industrial processes are scientifically sound, economically viable, and environmentally sustainable.
What challenges arise in applying the Laws of Chemical Combination to non-stoichiometric compounds?
Non-stoichiometric compounds, also known as berthollides, present challenges when applying the Laws of Chemical Combination because their composition does not follow simple whole-number ratios of elements. These compounds, often found in solid-state chemistry, have a variable composition due to defects in the crystal structure, such as vacancies or interstitial atoms.
For example, iron oxide (FeO) can exist with a composition that deviates from the ideal FeO formula, often written as Fe₀.₉₅O. This variability means that the Law of Definite Proportions does not strictly apply, as the ratio of iron to oxygen is not fixed.
In such cases, traditional stoichiometric principles must be adjusted to account for the varying composition. This can complicate the synthesis, analysis, and application of these materials. However, understanding the nature of these deviations is crucial in fields like materials science, where non-stoichiometric compounds play a significant role in the properties of ceramics, semiconductors, and catalysts.
How do the Laws of Chemical Combination apply to the concept of limiting reactants in chemical reactions?
The concept of limiting reactants is directly related to the Laws of Chemical Combination, particularly the Law of Conservation of Mass and stoichiometry. In a chemical reaction, the limiting reactant is the substance that is completely consumed first, determining the maximum amount of product that can be formed.
According to the Law of Conservation of Mass, the mass of the products is determined by the mass of the reactants, including the limiting reactant. Once the limiting reactant is consumed, the reaction cannot proceed, even if other reactants are still present.
For example, in the reaction between hydrogen and oxygen to form water:
2H₂ (g) + O₂ (g) → 2H₂O (g)
If you start with 5 moles of H₂ and 2 moles of O₂, the limiting reactant would be H₂ because it will be completely used up when it reacts with O₂ in the ratio given by the balanced equation. The Law of Definite Proportions ensures that the ratio of H₂ to O₂ remains constant, and the Law of Conservation of Mass ensures that the total mass of H₂O produced corresponds to the mass of H₂ and O₂ consumed.
Understanding limiting reactants is essential for predicting the amounts of products formed in chemical reactions and is a key concept in industrial chemistry, where maximizing product yield is crucial for efficiency and cost-effectiveness.